

These gaps are then filled by DNA polymerase, and thus the entire process of recombination is completed.įigure 2. Instead, both cut ends of the linear DNA sequence of mobile genetic element catalyze a direct attack on the target DNA, leaving two short single-stranded gaps in the recombinant DNA molecule, one at the 3′ end and the other at the 5′ end of the mobile genetic element. However, these integrases do not require specific DNA sequences in the target chromosome. Similar to λ integrase, each of these integrases recognizes a specific DNA sequence in the mobile genetic element that must be integrated into the target chromosome. In transpositional site-specific recombination, mobile DNA sequences encode integrases that insert their DNA into target chromosome by a mechanism different from that described for bacteriophage λ. Finally, the integrase dissociates from the DNA and is ready to be used for the next recombination reaction. Integrase then cuts the DNA section in both the bacteria and the bacteriophage and then, by using a short region of sequence homology, reseals the reaction.
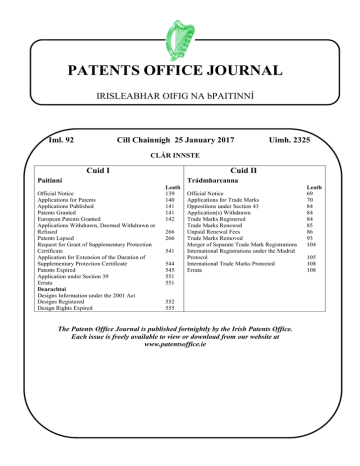
This DNA-integrase complex binds to a related but different specific sequence on the bacterial chromosome (target chromosome), bringing the bacterial and bacteriophage chromosomes close together. Several molecules of integrase protein bind to a specific DNA sequence of the circular bacteriophage chromosome (mobile genetic element). When a virus-in this case, bacteriophage λ-enters a cell, λ integrase is synthesized.
#INTRUSION 2 MAKU AND DNA CODE#
DNA sequences in the virus code for integrase in bacteriophage λ, it is termed λ integrase. The conservative site-specific recombination was first demonstrated in bacteriophage lambda (λ). 33,38–40 There are two types of site-specific recombination: conservative and transpositional. The homologous recombination does not normally change the rearrangement of the genes in a chromosome.īy contrast, site-specific recombination alters the relative positions of the nucleotide sequence in a chromosome, because DNA homology between the recombining DNA molecules is not required, and the pairing reaction depends on a recombination enzyme–mediated recognition of specific nucleotide sequences present on one or both recombining DNA molecules. The exchange of genetic material can occur anywhere in the homologous DNA sequences of two DNA helixes however, the mechanism of homologous recombination ensures that two regions of DNA double helix undergo an exchange reaction, provided that they have extensive sequence homology. 36,37 This exchange involves breaking of two homologous DNA double helixes and joining of the two broken ends, by base pairing, to their opposite partners (crossover) to create two intact DNA molecules, each composed of parts of the original DNA molecule. In homologous recombination an exchange of genetic material takes place between two pairs of homologous DNA sequences located on two copies of the same chromosome. There are two classes of genetic recombination: general, or homologous, and site-specific. 33–40 The mechanisms of genetic recombination allow large sections of DNA helix to move from one chromosome to another. To adapt to an ever-changing environment, DNA undergoes rearrangement, which is caused by genetic recombination. Wesley Burks MD, in Middleton's Allergy: Principles and Practice, 2020 DNA Rearrangement: Genetic Recombination
